Biomass power generation produces significant amounts of ash, the quantity of which varies depending on the type of biomass fuel and combustion technology employed. This study focuses on comparing ashes, with a particular emphasis on hazardous organic compounds, especially polycyclic aromatic hydrocarbons (PAHs). Ashes from wood or straw combustion are commonly used as fertilizers in agricultural soils. Samples were collected from power plants in the Czech Republic where wood chips, sawdust, bark, and straw were combusted. The organic fraction was isolated through extraction, and PAHs were determined using GC-UV. PAH concentrations ranged from 15 to 733 μg/kg. These compounds are formed during the pyrolysis stage of combustion through secondary aromatization reactions in char at temperatures above 400°C, and are bound in porous unburned carbon. GC-MS was used for a detailed qualitative analysis of other organic compounds, revealing higher and branched aliphatic hydrocarbons, ketones, amides, and phthalates in samples with higher unburned carbon content.
Industrial ashes are usually characterized by the inorganic components, physical features and unburned carbon. The organic compounds possibly present are not usually addressed. However, in the case of ashes from biomass combustion it is necessary to know not only the inorganic but also the organic harmful substances. The reason is that assessment the possibilities to utilize these ashes in agriculture is needed in the Czech Republic.
Analyses of the PAHs were conducted using the GC-UV and GC-MS methods. The actual analyses were preceded by extractions. Moreover, unburned carbon in ashes was determined and surface measurements were carried out. Preparation of the extract. The ash acquired from power plants was extracted such that 20 ml of a hexane-acetone solution (7:3) was added to 10 g of a dry sample with a grain size below 0.2 mm; the mix created was extracted by ultrasound for 30 minutes (Marvin et al., 1992; Sun et al., 2006) and subsequently shaken for 30 minutes on a shaker. Demineralized water was then added in the amount precisely needed for the separation of acetone. After preliminary analyses of both phases by the GC-UV method, the acquired n-hexane part of the extract was used for further analyses, because PAHs were not found in the water-acetone phase. The extract was not further purified. As needed, the extract was concentrated by a gentle stream of nitrogen. For quantitative analyses, the gas chromatography with ultraviolet detection (GCUV) was selected, which enables the compounds class analysis (Mostecký, 1984; Lagesson-Andrasko et al., 1998; Lagesson et al., 2000; Hatzinikolaou et al.,2006). The basis of the identification and determination of the aromatic and other organic compounds by GC-UV is that ultraviolet spectra of organic compounds in the gas phase are very well defined (Lagesson-Andrasko and Lagesson, 2005). Under appropriate conditions, the aromatics and PAHs could be monitored in the areas of UV wavelengths ~188-203 nm (single aromatic rings), ~210-229 (double aromatic rings) and ~240-259 nm (polyaromatics) (Lagesson et al., 2000). The class analysis of these hydrocarbons may be therefore successfully conducted in the mixture. Also further compounds, e.g. aliphatic hydrocarbons, ketones, aldehydes and others may be identified and determined. It is important that the agreement of the reference and analyzed spectra is very good even in very different concentrations. Further, the effect of the temperature of the detector on the shape of the UV spectrum is negligible.
Analyses were conducted on a gas chromatograph with an ultraviolet detector, Labio, a.s., Prague. The UV detector was a Quant UV spectrometer working after construction adjustments in the area 155-310 nm. (Short wavelengths in the range 155-190 nm are very important for the utilization of UV spectrometry in connection with gas chromatography, because a significant part of the absorption maxima is in the area of wavelengths shorter than 190 nm.) The spectrometer operating software was Specsoft HS, which was used for activation, treatment and evaluation of the UV data. This program was also used to display the spectra and acquired chromatographs. Separation of substances took place on a stainless packed column (240 cm x 2 mm) with a 3 % OV-1 fixed stationary phase on 100/120 Supelcoport (Supelco), thermally stable to 280 o C. The column was heated by electrical current. The GC oven was heated from 40 °C (2 min) to 260 °C (10 min) at a rate 20 °C/min. The temperature of UV detector was 260 °C. It did not have a perceptible influence on analytic results and was selected only considering the prevention of its contamination by condensation of separated compounds. The flow rate of carrier gas, ultra-clean nitrogen, was 50 ml/min. Peaks areas and PAHs contents were evaluated by Clarity Data Apex program. In acquired chromatograms the UV spectrum of the separated compound/s was acquired for each chromatographic peak. The identification was conducted following (Lagesson et al., 2000; Lagesson-Andrasko and Lagesson, 2005). The calibration was conducted with naphtalene, acenaphtylene, phenanthrene, fluoranthene and pyrene standards (Aldrich Chemical Comp., Inc.). By GC-UV the PAHs from naphtalenes to pyrenes as a prevailing lighter fraction of PAHs were identified and quantified.
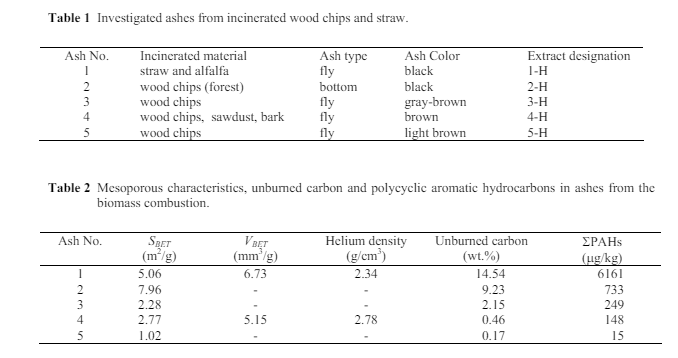
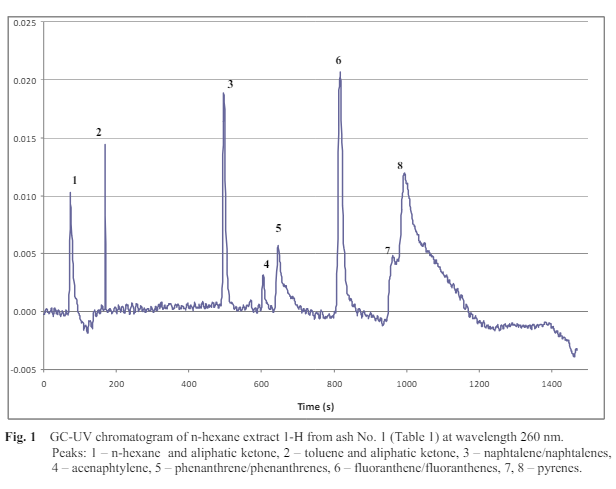
The ashes were formed under various operational conditions and contained different amounts of unburned carbon, had different inner surface area SBET, and contained different amounts of PAHs as GC-UV analyses showed. A typical GC-UV chromatogram of n-hexane extract is shown in Figure 1. Besides PAHs and toluene, aliphatic ketones were identified according to characteristic absorption triplet at 183-200 nm in UV spectra of peaks 1 and 2 (Lagesson et al., 2000). PAHs were found in all of the ashes, even in those whose content of unburned carbon was very low (Nos. 3-5, Table 2). It is known that in the course of the combustion of lignocelluloses the PAHs are created, but the question is how at the high temperatures of combustion these hydrocarbons remain in the resultant ash. It is likely that they are preserved in the porous system of the unburned carbon, which is a typical component of ash, because the process parameters of the combustion are never set ideally. The amount of unburned carbon in the ashes and their inner surface area SBET were therefore monitored as well. If this inner surface area grew with the amount of unburned carbon and at the same time with the unburned carbon also the PAHs content increased, it would probably mean that these hydrocarbons are preserved in the porous system of the unburned carbon. The results are summarized in Table 2. As expected, in considered samples the PAHs from naphtalenes to pyrenes were prevailing. But also benzo(a,e)pyrenes were identified by GC-MS in samples Nos. 1-3. Because of their hazardous potential, these compounds were quantified by GC-MS. Samples Nos. 1, 2, and 3 contained 382, 30, and 20 g/kg benzo(a,e)pyrenes, resp. These quantities are included in PAHs in Table 2.
Copyrigt: https://www.irsm.cas.cz/materialy/acta_content/2012_04/6.Straka_%20Havelcova.pdf